Genome sequencing and the diagnosis of novel coronavirus (SARS-COV-2) in Africa: how far are we?
Muki Shey, Joseph Chukwudi Okeibunor, Ali Ahmed Yahaya, Belinda Louise Herring, Oyewale Tomori, Sheick Omar Coulibaly, Hieronyma Nelisiwe Gumede-Moeletsi, Jason Mathiu Mwenda, Zabulon Yoti, Charles Shey Wiysonge, Ambrose Otau Talisuna
Corresponding author: Muki Shey, Welcome Centre for Infectious Disease Research in Africa (CIDRI-Africa), Institute of Infectious Disease and Molecular Medicine (IDM), University of Cape Town, Cape Town, South Africa
Received: 25 May 2020 - Accepted: 27 May 2020 - Published: 09 Jun 2020
Domain: Immunology,Infectious disease,Respiratory diseases
Keywords: RT-PCR, SARS-COV-2, COVID-19, serology test, antibody test, rapid diagnostic test (RDT), genome sequencing
©Muki Shey et al. Pan African Medical Journal (ISSN: 1937-8688). This is an Open Access article distributed under the terms of the Creative Commons Attribution International 4.0 License (https://creativecommons.org/licenses/by/4.0/), which permits unrestricted use, distribution, and reproduction in any medium, provided the original work is properly cited.
Cite this article: Muki Shey et al. Genome sequencing and the diagnosis of novel coronavirus (SARS-COV-2) in Africa: how far are we?. Pan African Medical Journal. 2020;36:80. [doi: 10.11604/pamj.2020.36.80.23723]
Available online at: https://www.panafrican-med-journal.com/content/article/36/80/full
Review 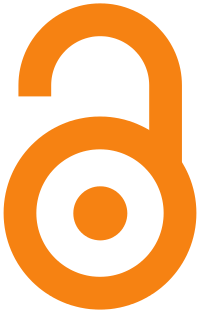
Genome sequencing and the diagnosis of novel coronavirus (SARS-COV-2) in Africa: how far are we?
Genome sequencing and the diagnosis of novel coronavirus (SARS-COV-2) in Africa: how far are we?
Muki Shey1,2,&, Joseph Chukwudi Okeibunor3, Ali Ahmed Yahaya3, Belinda Louise Herring3, Oyewale Tomori3, Sheick Omar Coulibaly3, Hieronyma Nelisiwe Gumede-Moeletsi3, Jason Mathiu Mwenda3, Zabulon Yoti3, Charles Shey Wiysonge4,5, Ambrose Otau Talisuna3
1Welcome Centre for Infectious Disease Research in Africa (CIDRI-Africa), Institute of Infectious Disease and Molecular Medicine (IDM), University of Cape Town, Cape Town, South Africa, 2Department of Medicine, University of Cape Town, Cape Town, South Africa, 3World Health Organization (WHO), Africa Office, Brazzaville, Congo, 4Cochrane Centre, South African Medical Research Council, Cape Town, South Africa, 5School of Public Health and Family Medicine, University of Cape Town, Cape Town, South Africa
&Corresponding author
Muki Shey, Welcome Centre for Infectious Disease Research in Africa (CIDRI-Africa), Institute of Infectious Disease and Molecular Medicine (IDM), University of Cape Town, Cape Town, South Africa
The coronavirus disease (COVID-19) caused by the novel severe acute respiratory syndrome coronavirus 2 (SARS-COV-2) has become a pandemic. There is currently no vaccine or effective treatment for COVID-19. Early diagnosis and management is key to favourable outcomes. In order to prevent more widespread transmission of the virus, rapid detection and isolation of confirmed cases is of utmost importance. Real time reverse transcriptase polymerase chain reaction (RT-PCR) is currently the "gold standard" for the detection of SARS-COV-2. There are several challenges associated with this test from sample collection to processing and the longer turnaround time for the results to be available. More rapid and faster diagnostic tests that may produce results within minutes to a few hours will be instrumental in controlling the disease. Serological tests that detect specific antibodies to the virus may be such options. In this review, we extensively searched for studies that compared RT-PCR with serological tests for the diagnosis of COVID-19. We extracted the data from the various selected studies that compared the different tests and summarised the available evidence to determine which test is more appropriate especially in Africa. We also reviewed the current evidence and the challenges for the genome sequencing of SARS-COV-2 in Africa. Finally, we discuss the relevance of the different diagnostic tests and the importance of genome sequencing in identifying potential therapeutic options for the control of COVID-19 in Africa.
Since being detected in Wuhan, China, in December 2019, the novel severe acute respiratory syndrome coronavirus 2 (SARS-COV-2) has spread to 216 countries, areas or territories worldwide including 54 African countries where more than 95,000 cases have been confirmed with over 2,950 deaths and 34,000 recoveries [1]. SARS-COV-2 is the etiologic agent for the coronavirus disease (COVID-19), which is now a pandemic. Real time reverse transcription polymerase chain reaction (RT-PCR) is currently the test for the detection of SARS-COV-2 in human samples derived from the lower or upper respiratory tract [2]. Positive tests using blood and anal swabs have also been reported [3,4]. Swabs or fluid is used for the isolation of genetic material, RNA, which is reversed transcribed to cDNA and amplified in the presence of specific reagents (such as primers, probes), if the virus was present in the clinical samples. In the absence of the virus, no viral RNA will be present and consequently no amplification.
This test requires trained personnel for good sample collection and extraction of the RNA to avoid false negative results (or false positive results if contamination occurs) [5]. Appropriate laboratory infrastructure including biosafety cabinets, personal protective equipment (PPE) and instruments for the extraction, running and analysis of the results are also required [6]. RT-PCR is currently the “gold standard” for the detection of active infection. However, clinical indicators should also be taken into consideration for the diagnosis, treatment, management and isolation of patients [5]. The average turnaround time for RT-PCR diagnostic test may range from one to several days, in resource-limited settings. This test may be relevant for hospitalised patients but can be a challenge for mass screening or in cases where results are needed immediately for treatment and isolation of infected individuals. More rapid and cheaper detection tests that do not require highly trained personnel and complex infrastructure are needed to scale up testing especially in less-resourced environments. Serological tests that detect specific antibodies against the virus may offer such alternatives, but they are also associated with some challenges [6-8].
Since the beginning of the SARS-COV-2 outbreak, the World Health Organisation (WHO) has provided training to atleast 44 African countries who now have the capacity to perform RT-PCR testing to detect the virus [1]. Africa Centre for Disease Control and Prevention (Africa CDC) is also instrumental in supporting member states with reagents and equipment to facilitate testing for SARS-COV-2 [9]. In this review, we aimed to evaluate studies comparing laboratory testing by RT-PCR to other detection tests, mainly serology tests for antibodies against SARS-COV-2 (including the rapid diagnostic tests, RDTs) and their relevance in the fight against COVID-19 in Africa. We also summarise evidence for the SARS-COV-2 genome sequencing in Africa and the importance in the context of global data.
We performed a search of peer-reviewed published articles indexed mainly on PubMed (28th April 2020) for the diagnosis of SARS-COV-2 (Table 1). We also searched MedRxiv and BioRxiv for publicly available articles that may not yet have been peer-reviewed. Reference lists of published articles (including reviews) and documents on databases such as WHO, CDC and GISAID were also scanned for potential articles.
We went through the search result articles and selected either the primary research articles or meta-analyses of articles that compared two or more tests for the detection of SARS-COV-2. Normal review articles or personal communication or articles reporting only treatment strategies (without a virus detection component) were not included in the summary, but some critical ones were referenced where necessary. Only articles reporting data (complete or partial) in English were selected. We categorized articles that compared RT-PCR and any antibody test (26 articles) (Table 2) [4,10-34], or articles that compared RT-PCR with any test that was described as “rapid” or “point-of-care” (8 articles) [35-42], for the detection of SARS-COV-2 (Table 2). The 26 articles comparing RT-PCR with serology test were geographically distributed as follows: Mainland China (20), Finland (1), Netherlands (1), France (1), Peru (1), Germany (1) and Hong Kong (1). The 8 articles comparing RT-PCR and rapid tests were from Mainland China (4), Italy (1), Germany (1), Brazil (1; meta-analysis) and Taiwan (1). A further 4 articles were found that describe the detection of neutralizing antibodies against SARS-COV-2 with no direct comparison with RT-PCR, mainly for therapeutic purposes and not discussed in this review. These were from Mainland China (2), USA (1) and Singapore (1) [43-46].
RT-PCR and serology antibody tests to detect SARS-COV-2: RT-PCR was used as the gold standard for detecting SARS-COV-2 and the antibody tests were compared to the RT-PCR test. All studies evaluated either immunoglobulin M (IgM) or IgG or both that are specific for SARS-COV-2. There was a wide variation between studies in the number of participants included or those who underwent molecular (and serology) testing for SARS-COV-2, ranging from 1 participant (five case reports) to 951 participants (though not all had both tests performed) (Table 2). The agreement between the RT-PCR and the antibody detection tests ranged from 100% to below 20%. The antibodies against SARS-COV-2 were detected from as early as 2 days post onset of symptoms to as late as 7 weeks or more post onset of symptoms. With a few exceptions that used plasma alone, most studies evaluated the antibodies using serum (sometime with plasma and whole blood as well) that was collected from participants at various times from admission to several weeks post recovery or discharge from hospitals (for hospitalized patients). The current wide range in the detection period (time to seroconversion) for the antibodies make them less useful as diagnostic tests for acute infection, but still very useful as supporting tests in determining previous infection (whether still an active infection or not) with SARS-COV-2. Further development and (independent) validation of these antibody tests is required. The CDC has designed and validated a serologic test which is currently being used to guide the response to the COVID-19 pandemic in the United States and they are also testing several commercially available serologic tests and results are expected soon [7].
Even though these were case reports and prone to reporting bias, two studies (1 participant each) reported data on COVID-19 in individuals co-infected with HIV (and one with HCV) [12,25]. Both studies reported delayed seroconversion and antibody detection, 4-7 weeks from onset of symptoms. One study reported persistent negative RT-PCR results [25], while the second reported one positive RT-PCR out of 4 serial tests [12]. If these are confirmed in larger powered studies, it would suggest antibody testing might be more relevant in the diagnosis of COVID-19 in HIV-infected participants. Currently no other studies have reported evaluation of the diagnosis of SARS-COV-2 in HIV-infected patients, especially those with advanced disease who are usually at high risk of developing other infectious diseases and complications [47,48]. One study evaluated diagnosis of SARS-COV-2 in a pregnant woman [17]. The woman tested positive for RT-PCR and antibodies and 16 hours after giving birth (by caesarian section), the newborn also tested positive for SARS-COV-2 by RT-PCR but did not have detectable antibodies. If confirmed in larger powered studies, this may have implications for vertical transmission of SARS-COV-2.
Rapid diagnostic tests for the detection of SARS-COV-2: several rapid diagnostic tests (RDTs) have been developed and tested in single studies but none has been independently validated and commercially available for global use. Most of these tests have been developed and tested in Mainland China. Chen et al. described the development and testing of a lateral flow immunoassay (LFIA) that could detect SARS-COV-2-specific antibodies in serum within 10 minutes. This test detected antibodies in all 7 samples that were RT-PCR positive and 1 (out of 12) sample that was RT-PCR negative but clinically suspicious of SARS-COV-2 [38]. Yong et al. compared the diagnostic potential of RT-PCR and IgG/IgM antibody test for SARS-COV-2 and found that the RT-PCR was great at detecting early infection (92% of suspected COVID-19 cases (n=38) while antibody detection ranged from 23% (IgM) to 53% (IgG)) [42]. Antibody tests performed better at detecting prolonged infection (>7 days post symptom onset, ranging from 50% (IgM) to 92% (IgG). Li et al. evaluated rapid antibody test that could detect SARS-COV-2 antibodies in blood within 15 minutes and showed that 256 of 397 RT-PCR confirmed COVID-19 cases had detectable IgM-IgG antibodies [40]. Pan et al. used whole blood, serum and plasma samples to evaluate the rapid detection of SARS-COV-2 in RT-PCR confirmed samples and showed variation in agreement between the antibody test and RT-PCR ranging from 4-100% depending on the severity of the disease and timing of the test; the agreement was low within 7 days and highest around 14 days post onset of symptoms [39].
RDTs have also been developed and tested in other countries other than Mainland China. In Taiwan, Lee et al. tested 14 samples that were positive for RT-PCR using a rapid LFIA IgG/IgM antibody test kit which detected antibodies in 100% of the RT-PCR positive symptomatic patients and no antibodies in the RT-PCR positive asymptomatic patients and these antibodies were detected between 5 to 42 days post onset of symptoms [36]. In Italy, Spicuzza et al. compared 30 individuals, with 23 RT-PCR positive and 7 RT-PCR negative and showed that 19/23 were IgM/IgG positive (at day 9) using a 15-minute rapid test with whole blood, while only 1/7 RT-PCR negative was positive for the IgM/IgG at day 18 [35]. In Germany, Dohla et al. described an IgG/IgM-based rapid point-of-care (POI) test using blood drops that showed very low sensitivity of 36% and high specificity of 89% [37]. In Brazil, Castro et al. in a meta-analysis evaluating the different rapid antibody test kits and RT-PCR showed a 94% positive agreement between RT-PCR and serology tests where 120 participants tested positive for the IgG/IgM antibodies out of 128 who were RT-PCR positive [41].
Based on the above information, molecular testing by RT-PCR remain the main diagnostic test for early detection of SARS-COV-2 and antibody tests may play a secondary supportive role to indicate immune responses to the virus. However, if a rapid antibody test (or any other test type) with high sensitivity (and specificity) is developed and validated that could detect an active infection within minutes, this will have important implications in the early detection and treatment of patients with SARS-COV-2. The United States´ Food and Drug Administration (FDA) recently approved another molecular test (Xpert® Xpress SARS-COV-2) developed by cepheid for emergency use only, which can produce results in less than an hour from sample collection [49]. Eventhough hands-on sample processing of swabs after collection is minimal, expensive equipment are still needed to perform the test, making it less suitable for mass screening in resource-limited settings (Table 2, Table 2 (suite)).
Genome sequencing of the SARS-COV-2 strains in Africa: as of 21st May 2020, there were 186 SARS-COV-2 genome sequences that have been completed in eight African countries (out of more than 30,000 sequences globally), as follows: Democratic Republic of Congo, DRC (124), Senegal (22), Ghana (14), South Africa (17), Algeria (3), Gambia (3), Egypt (2) and Nigeria (1) [50-52]. This suggests that though there is progress, from the first published sequenced in Nigeria on March 6, 2020 [52], Africa still needs to up its game and build more capacity with respect to genome sequencing. Understanding sequence distribution of the SARS-COV-2 strains circulating on the continent would help in understanding transmission dynamics and could eventually assist in the development of vaccine against COVID-19. The African sequences are relatively widely distributed within the global sequences [50]. This suggests importation of virus from different regions of the world. This may also suggest that effective vaccines that may be developed and tested in other parts of the world may also work well within Africa and there may be no need for continent-specific vaccines. However, vaccine testing in Africa is still strongly recommended.
SARS-COV-2 sequencing and diagnosis (implications for Africa): of all the studies on the various diagnostic and antibody detection tests identified and discussed in this review, none was conducted in Africa. This may be as a result of most African countries still largely focusing on early detection (by RT-PCR of SARS-COV-2) and treatment to control and prevent the pandemic taking hold in Africa. In addition, most of the ongoing research studies we are aware of on the African continent are focused on comparing treatment options and management of COVID-19 patients rather than evaluating diagnostic tests, as the RT-PCR is still the test of choice. There are reports that there are already a few LFIA tests developed in South Africa that are currently being tested, but results are not yet available [6]. The findings from the 2 case reports that COVID-19 clinically-diagnosed patients with HIV had persistent RT-PCR negative tests and delayed seroconversion from onset of symptoms might have clinical implications for the diagnosis and management of COVID-19 patients with HIV co-infection in Africa given that most African countries have high prevalence of HIV. These data however should be interpreted with caution. Africa is also contributing to the genome data with 8 countries so far (as of 21st May 2020) reporting data on genome sequencing [50-52]. This is commendable but more countries and governments, with the support of international organisations such as the WHO, will need to support and encourage more collection and sequencing of the various circulating strains on the continent. Institutions such as the pathogens genomics intelligence institute (PGII) of the Africa CDC will be instrumental in facilitating the sequencing of genomes for SARS-COV-2 and other pathogens and strengthening health systems and institutions for improved prevention, detection and response to public health threats on the continent and beyond [53]. COVID-19 pandemic has also presented the opportunity for African researchers in science and technology to collaborate more and pull resources together to increase research on COVID-19 and other infectious diseases in Africa [54]. For example, researchers may be able to share information or collaborate so that those with more resources could assist in sequencing the strains from countries with less resources. DRC has contributed more than 66% of the SARS-COV-2 genome sequences from Africa likely due to the established infrastructure from the Ebola virus response. Other African countries may leverage this available infrastructure to sequence more genomes.
Even though we performed a comprehensive search for articles to include in this review, the search was not exhaustive as we did not search all available databases. We are confident that even if we missed any studies, the evidence will not be drastically different from that presented in this review. Because RT-PCR was used as the gold-standard, we limited our selected articles to only studies that performed RT-PCR in addition to the serology test for comparison purposes and studies that performed RT-PCR alone or serology test alone were not considered.
As SARS-COV-2 infections continue to rise on the continent, Africa will need to join the rest of the world in the development and testing of new diagnostic methods. Further research on the diagnosis of SARS-COV-2 in patients with infectious and non-communicable diseases (such as HIV, tuberculosis, diabetes) and other potential at-risk patient groups (such as pregnant women) is also needed to understand the relationship between comorbidities and COVID-19 pathogenesis as well as the effect of COVID-19 on the pathogenesis of other diseases and vice versa. In addition, more genome sequencing of African strains, building collaborations between African researchers and development of capacity to complete genome sequencing in most African countries should be encouraged and supported.
What is known about this topic
- The challenges associated with the different diagnostic tests for SARS-COV-2, but limited data on direct comparisons of the different tests;
- Limited infrastructure for mass testing and sequencing of SARS-COV-2.
What this study adds
- A detailed review of available evidence on genome sequencing in Africa, the challenges and the opportunities;
- A comprehensive synthesis of available evidence for the laboratory diagnosis of SARS-COV-2, direct comparisons between the performances of the different tests, research and development needs for the different tests in Africa, the challenges and the opportunities;
- Discussions and reflections on the state of genome sequencing and mass screening of SARS-COV-2 in Africa, and future directions.
The authors declare no competing interests.
JCO, AAY, BLH, OT, SOC, HNG, JMM, ZY, and AOT conceived and commissioned the study. MS performed the literature search and screened for the selected studies, extracted the data and wrote the first draft of the manuscript. CW supervised all aspects of the study. All the authors have read and agreed to the final manuscript.
We thank Nyaradzai Mitchell Chimukuche who assisted with data extraction and reviewed the first draft of the manuscript. Muki Shey receives funding from the Wellcome Trust (Grant#: 211360/Z/18/Z) and the National Research Foundation (NRF) of South Africa (Grant#:UID127558).
Table 1: search terms and number of studies identified
Table 2: studies comparing the use of molecular and serology assays for the detection of SARS-COV-2 with key findings from each study
Table 2 (suite): studies comparing the use of molecular and serology assays for the detection of SARS-COV-2 with key findings from each study
- WHO. African countries move from COVID-19 readiness to response as many confirm cases. 2020. Accessed on 21 May 2020.
- CDC. Interim guidelines for collecting, handling and testing clinical specimens from persons for coronavirus disease 2019 (COVID-19). 2020. Accessed on 29 April 2020.
- Liu J, Xiao Y, Shen Y, Shi C, Chen Y, Shi P et al. Detection of SARS-CoV-2 by RT-PCR in anal from patients who have recovered from coronavirus disease 2019. J Med Virol. 2020 Apr 14;10.1002/jmv.25875. PubMed | Google Scholar
- Zhang W, Du RH, Li B, Zheng XS, Yang XL, Hu B et al. Molecular and serological investigation of 2019-nCoV infected patients: implication of multiple shedding routes. Emerg Microbes Infect. 2020;9(1):p. 386-389. PubMed | Google Scholar
- Li Y, Yao L, Li J, Chen L, Song Y, Cai Z et al. Stability issues of RT-PCR testing of SARS-CoV-2 for hospitalized patients clinically diagnosed with COVID-19. J Med Virol. 2020 Mar 26;10.1002/jmv.25786. PubMed | Google Scholar
- Dheda K, Jaumdally S, Davids M, Chang JW, Jina P, Pooran A et al. Diagnosis of COVID-19: considerations, controversies and challenges. Afr J Thoracic Crit Care Med. 2020;23(2). Google Scholar
- CDC. Serology testing for COVID-19 at CDC. 2020. Accessed on 1st May 2020.
- Adebisi YA, Oke GI, Ademola PS, Chinemelum IG, Ogunkola IO, Lucero-Prisno III DE. SARS-CoV-2 diagnostic testing in Africa: needs and challenges. Pan African Medical Journal. 2020 April 14;35(2):4. Google Scholar
- Africa-CDC. Outbreak Brief 15: COVID-19 Pandemic-28 April 2020. 2020. Accessed on 28 April 2020.
- Qu J, Wu C, Li X, Zhang G, Jiang Z, Li X et al. Profile of IgG and IgM antibodies against severe acute respiratory syndrome coronavirus 2 (SARS-CoV-2). Clin Infect Dis. 2020 Apr 27;ciaa489. PubMed | Google Scholar
- Lou B, Li T, Zheng S, Su Y, Li Z, Liu W et al. Serology characteristics of SARS-CoV-2 infection since the exposure and post symptoms onset. medRxiv. 2020;2000763. PubMed
- Wang M, Luo L, Bu H, Xia H. Case report: one case of coronavirus disease 2019(COVID-19) in patient co-nfected by HIV with a low CD4+ T cell count. Int J Infect Dis. 2020 Apr 23;96:148-150. PubMed | Google Scholar
- Xie J, Ding C, Li J, Wang Y, Guo H, Lu Z et al. Characteristics of patients with coronavirus disease (COVID-19) confirmed using an IgM-IgG antibody test. J Med Virol. 2020 April 24. PubMed | Google Scholar
- Yang JR, Deng DT, Wu N, Yang B, Li HJ, Pan XB. Persistent viral RNA positivity during recovery period of a patient with SARS-CoV-2 infection. J Med Virol. 2020 April 24. PubMed | Google Scholar
- Yongchen Z, Shen H, Wang X, Shi X, Li Y, Yan J et al. Different longitudinal patterns of nucleic acid and serology testing results based on disease severity of COVID-19 patients. Emerg Microbes Infect. 2020;9(1):833-836. PubMed | Google Scholar
- Xiang F, Wang X, He X, Peng Z, Yang B, Zhang J et al. Antibody detection and dynamic characteristics in patients with COVID-19. Clin Infect Dis. 2020 Apr 19;ciaa461. PubMed | Google Scholar
- Alzamora MC, Paredes T, Caceres D, Webb CM, Valdez LM, La Rosa M. Severe COVID-19 during pregnancy and possible vertical transmission. Am J Perinatol. 2020 Apr 18. PubMed | Google Scholar
- Lin D, Liu L, Zhang M, Hu Y, Yang Q, Guo J et al. Evaluation of serological tests in the diagnosis of 2019 novel coronavirus (SARS-CoV-2) infections during the COVID-19 outbreak. medRxiv. 2020. Google Scholar
- Zhao J, Yuan Q, Wang H, Liu W, Liao X, Su Y et al. Antibody responses to SARS-CoV-2 in patients of novel coronavirus disease 2019. Clin Infect Dis. 2020 Mar 28;ciaa344. PubMed | Google Scholar
- Zhong L, Chuan J, Gong B, Shuai P, Zhou Y, Zhang Y et al. Detection of serum IgM and IgG for COVID-19 diagnosis. Sci China Life Sci. 2020;63(5):p. 777-780. PubMed | Google Scholar
- Okba NMA, Müller MA, Li W, Wang C, Geurtsvan Kessel CH, Corman VM et al. Severe acute respiratory syndrome coronavirus 2-specific antibody responses in coronavirus disease 2019 patients. Emerg Infect Dis. 2020;26(7). PubMed | Google Scholar
- Jin Y, Wang M, Zuo Z, Fan C, Ye F, Cai Z et al. Diagnostic value and dynamic variance of serum antibody in coronavirus disease 2019. Int J Infect Dis. 2020;94:p. 49-52. PubMed | Google Scholar
- Liu W, Liu L, Kou G, Zheng Y, Ding Y, Ni W et al. Evaluation of nucleocapsid and spike protein-based ELISAs for detecting antibodies against SARS-CoV-2. J Clin Microbiol. 2020 Mar 30;58(6):e00461-20. PubMed | Google Scholar
- Guo L, Ren L, Yang S, Xiao M, Chang D, Yang F et al. Profiling early humoral response to diagnose novel coronavirus disease (COVID-19). Clin Infect Dis. 2020 Mar 21;ciaa310. PubMed | Google Scholar
- Zhao J, Liao X, Wang H, Wei L, Xing M, Liu L et al. Early virus clearance and delayed antibody response in a case of COVID-19 with a history of co-infection with HIV-1 and HCV. Clin Infect Dis. 2020 Apr 9;ciaa408. PubMed | Google Scholar
- To KK, Tsang OT, Leung WS, Tam AR, Wu TC, Lung DC et al. Temporal profiles of viral load in posterior oropharyngeal saliva samples and serum antibody responses during infection by SARS-CoV-2: an observational cohort study. Lancet Infect Dis. 2020 May;20(5):565-574. PubMed | Google Scholar
- Xiao AT, Gao C, Zhang S. Profile of specific antibodies to SARS-CoV-2: the first report. J Infect. 2020 Mar 21;S0163-4453(20):30138-9. PubMed | Google Scholar
- Haveri A, Smura T, Kuivanen S, Österlund P, Hepojoki J, Ikonen N et al. Serological and molecular findings during SARS-CoV-2 infection: the first case study in Finland, January to February 2020. Euro Surveill. 2020;25(11):2000266. PubMed | Google Scholar
- Wölfel R, Corman VM, Guggemos W, Seilmaier M, Zange S, Müller MA et al. Virological assessment of hospitalized patients with COVID-2019. Nature. 2020 Apr 1;581(7809):465-469. PubMed | Google Scholar
- Li Z, Yi Y, Luo X, Xiong N, Liu Y, Li S et al. Diagnostic indexes of a rapid IgG/IgM combined antibody test for SARS-CoV-2. medRxiv. 2020;10.1002/jmv.25727. PubMed | Google Scholar
- Zhang P, Gao Q, Wang T, Ke Y, Mo F, Jia R et al. Evaluation of recombinant nucleocapsid and spie protein serological diagnosis of novel coronavirus disease 2019 (COVID-19). medRxiv. 2020. Google Scholar
- Grzelak L, Temmam S, Planchais C, Demeret C, Huon C, Guivel F et al. SARS-CoV-2 serological analysis of COVID-19 hospitalized patients, pauci-symptomatic individuals and blood donors. medRxiv. 2020. Google Scholar
- Long Q, Deng H, Chen J, Hu J, Liu B, Liao P et al. Antibody responses to SARS-CoV-2 in COVID-19 patients: the perspective application of serological tests in clinical practice. medrvix. 2020. Google Scholar
- Yong G, Yi Y, Tuantuan L, Xiaowu W, Xiuyong L, Ang L et al. Evaluation of the auxiliary diagnostic value of antibody assays for the detection of novel coronavirus (SARS-CoV-2). J Med Virol. 2020 Apr 22. PubMed | Google Scholar
- Spicuzza L, Montineri A, Manuele R, Crimi C, Pistorio MP, Campisi R et al. Reliability and usefulness of a rapid IgM-IgG antibody test for the diagnosis of SARS-CoV-2 infection: a preliminary report. J Infect. 2020 Apr 23:S0163-4453(20):30231-0. PubMed | Google Scholar
- Lee YL, Liao CH, Liu PY, Cheng CY, Chung MY, Liu CE et al. Dynamics of anti-SARS-Cov-2 IgM and IgG antibodies among COVID-19 patients. J Infect. 2020 Apr 23;S0163-4453(20):30230-9. PubMed | Google Scholar
- Döhla M, Boesecke C, Schulte B, Diegmann C, Sib E, Richter E et al. Rapid point-of-care testing for SARS-CoV-2 in a community screening setting shows low sensitivity. Public Health. 2020;182:p. 170-172. PubMed | Google Scholar
- Chen Z, Zhang Z, Zhai X, Li Y, Lin L, Zhao H et al. Rapid and sensitive detection of anti-SARS-CoV-2 IgG using lanthanide-doped nanoparticles-based lateral flow immunoassay. Anal Chem. 2020 May 19;92(10):7226-7231. PubMed | Google Scholar
- Pan Y, Li X, Yang G, Fan J, Tang Y, Zhao J et al. Serological immunochromatographic approach in diagnosis with SARS-CoV-2 infected COVID-19 patients. J Infect. 2020 Apr 10:S0163-4453(20):30175-4. PubMed | Google Scholar
- Li Z, Yi Y, Luo X, Xiong N, Liu Y, Li S et al. Development and clinical application of a rapid IgM-IgG combined antibody test for SARS-CoV-2 infection diagnosis. J Med Virol. 2020 Feb 27;10.1002/jmv.25727. PubMed | Google Scholar
- Castro R, Luz PM, Wakimoto MD, Veloso VG, Grinsztejn B, Perazzo H. COVID-19: a meta-analysis of diagnostic test accuracy of commercial assays registered in Brazil. Braz J Infect Dis. 2020;24(2):180-187. PubMed | Google Scholar
- Poh CM, Carissimo G, Wang B, Amrun SN, Lee CY, Chee RS et al. Potent neutralizing antibodies in the sera of convalescent COVID-19 patients are directed against conserved linear epitopes on the SARS-CoV-2 spike protein. bioRxiv. 2020. Google Scholar
- Ju B, Zhang Q, Ge X, Wang R, Yu J, Shan S et al. Potent human neutralizing antibodies elicited by SARS-CoV-2 infection. bioRxiv. 2020. PubMed | Google Scholar
- Wu F, Wang A, Liu M, Wang Q, Chen J, Xia S et al. Neutralizing antibody responses to SARS-CoV-2 in a COVID-19 recovered patient cohort and their implications. medRxiv. 2020. PubMed | Google Scholar
- Amanat F, Stadlbauer D, Strohmeier S, Nguyen T, Chromikova V, McMahon M et al. A serological assay to detect SARS-CoV-2 seroconversion in humans. medRxiv. 2020. PubMed | Google Scholar
- De Francesco D, Verboeket SO, Underwood J, Bagkeris E, Wit FW, Mallon PWG et al. Patterns of co-occurring comorbidities in people living with HIV. Open Forum Infect Dis. 2018;5(11):p. ofy272. PubMed | Google Scholar
- Schutz C, Barr D, Andrade BB, Shey M, Ward A, Janssen S et al. Clinical, microbiologic and immunologic determinants of mortality in hospitalized patients with HIV-associated tuberculosis: a prospective cohort study. PLoS Med. 2019;16(7):e1002840. PubMed | Google Scholar
- Cepheid. Xpert® Xpress SARS-CoV-2 has received FDA Emergency Use Authorization. A rapid, near-patient test for the detection of the 2019 novel coronavirus that causes COVID-19. 2020. Accessed on 11 May 2020.
- GISAID. Genomic epidemiology of hCoV-19. 2020. Accessed on 2 May 2020.
- Allam M. Whole-genome sequence of the severe acute respiratory syndrome coronavirus 2 (SARS-CoV-2) obtained from a South African coronavirus disease 2019 (COVID-19) patient. 2020. Accessed on 2 May 2020.
- Oluniyi P. First African SARS-CoV-2 genome sequence from Nigerian COVID-19 case. 2020. Accessed on 2 May 2020.
- Africa-CDC. Africa CDC Pathogen Genomics Intelligence Institute. 2020. Accessed on 2 May 2020.
- Maswime S, Dandara C, Sivarasu S. Coronavirus: never been a more compelling time for African scientists to work together. 2020. Accessed on 2 May 2020.
- Yong SEF, Anderson DE, Wei WE, Pang J, Chia WN, Tan CW et al. Connecting clusters of COVID-19: an epidemiological and serological investigation. Lancet Infect Dis. 2020 Apr 21:S1473-3099(20):30273-5. PubMed | Google Scholar